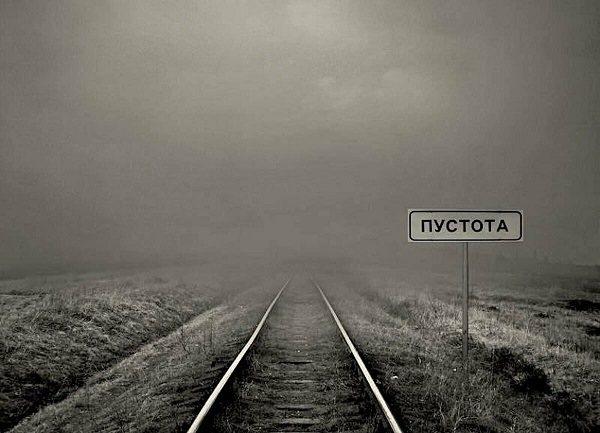
So that emptiness ceases to be emptiness
A vacuum is a place where, even if you don't see it, a lot happens. However, to find out what exactly requires so much energy that until recently it seemed impossible for scientists to look into the world of virtual particles. When some people stop in such a situation, it is impossible for others to encourage them to try.
According to quantum theory, empty space is filled with virtual particles that pulsate between being and non-being. They're also completely undetectable - unless we had something powerful to find them.
"Usually, when people talk about a vacuum, they mean something that's completely empty," said the theoretical physicist Mattias Marklund of the Chalmers University of Technology in Gothenburg, Sweden, in the January issue of NewScientist.
It turns out that the laser can show that it's not that empty at all.
Electron in a statistical sense
Virtual particles are a mathematical concept in quantum field theories. They are physical particles that manifest their presence through interactions, but violate the principle of the shell of the mass.
Virtual particles appear in the works of Richard Feynman. According to his theory, each physical particle is in fact a conglomerate of virtual particles. A physical electron is actually a virtual electron emitting virtual photons, which decay into virtual electron-positron pairs, which in turn interact with virtual photons - and so on endlessly. The "physical" electron is an ongoing process of interaction between virtual electrons, positrons, photons, and possibly other particles. The "reality" of an electron is a statistical concept. It is impossible to say which part of this set is really real. It is only known that the sum of the charges of all these particles results in the charge of the electron (i.e., to put it simply, there must be one more virtual electron than there are virtual positrons) and that the sum of the masses of all the particles creates the mass of the electron.
Electron-positron pairs are formed in the vacuum. Any positively charged particle, e.g. a proton, will attract these virtual electrons and repel positrons (with the help of virtual photons). This phenomenon is called vacuum polarization. Electron-positron pairs rotated by a proton
they form small dipoles which change the field of the proton with their electric field. The electric charge of the proton we measure is therefore not that of the proton itself, but of the entire system, including the virtual pairs.
A laser into a vacuum
The reason we believe that virtual particles exist goes back to the foundations of quantum electrodynamics (QED), a branch of physics that tries to explain the interaction of photons with electrons. Since this theory was developed in the 30s, physicists have been wondering how to deal with the problem of particles that are mathematically necessary but cannot be seen, heard or felt.
The QED shows that theoretically, if we create a sufficiently strong electric field, then the virtual accompanying electrons (or making up a statistical conglomerate called an electron) will reveal their presence and it will be possible to detect them. The energy required for this must reach and exceed the limit known as the Schwinger limit, beyond which, as it is figuratively expressed, the vacuum loses its classic properties and ceases to be "empty". Why is it not that simple? According to the assumptions, the required amount of energy must be as much as the total energy produced by all power plants in the world - another billion times.
The thing seems beyond our reach. As it turns out, however, not necessarily if one uses the laser technique of ultra-short, high-intensity optical pulses, developed in the 80s by last year's Nobel Prize winners, Gérard Mourou and Donna Strickland. Mourou himself openly said that the giga-, tera-, and even petawatt powers achieved in these laser supershots create an opportunity to break the vacuum. His concepts were embodied in the Extreme Light Infrastructure (ELI) project, supported by European funds and developed in Romania. There are two 10-petawatt lasers near Bucharest that scientists want to use to overcome the Schwinger limit.
However, even if we manage to break the energy limitations, the result - and what will eventually appear to the eyes of physicists - remains highly uncertain. In the case of virtual particles, the research methodology begins to fail, and the calculations no longer make sense. A simple calculation also shows that the two ELI lasers generate too little energy. Even four combined bundles are still 10 times less than necessary. However, scientists are not discouraged by this, because they consider this magic limit not a sharp one-off limit, but a gradual area of change. So they hope for some virtual effects even with smaller doses of energy.
Researchers have various ideas on how to strengthen the laser beams. One of them is the rather exotic concept of reflecting and amplifying mirrors that travel at the speed of light. Other ideas include amplifying the beams by colliding photon beams with electron beams, or colliding laser beams, which scientists at the Chinese Station of Extreme Light research center in Shanghai are said to want to carry out. A great collider of photons or electrons is a new and interesting concept that is worth observing.
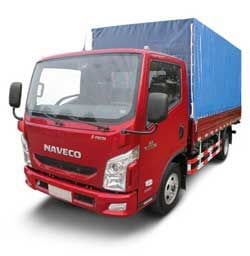
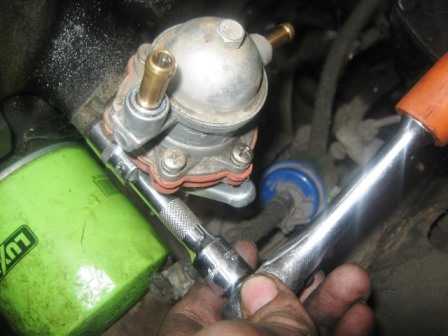