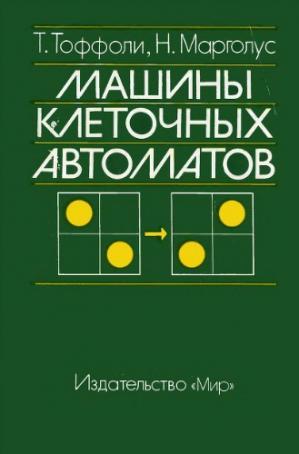
Cell machines
In 2016, the Nobel Prize in Chemistry was awarded for an impressive achievement - the synthesis of molecules that act as mechanical devices. However, it cannot be said that the idea of creating miniature machines is an original human idea. And this time nature was first.
The awarded molecular machines (more about them in the article from the January issue of MT) are the first step towards a new technology that may soon turn our lives upside down. But the bodies of all living organisms are full of nanoscale mechanisms that keep cells functioning efficiently.
In the center…
... cells contain a nucleus, and genetic information is stored in it (bacteria do not have a separate nucleus). The DNA molecule itself is amazing - it consists of more than 6 billion elements (nucleotides: nitrogenous base + deoxyribose sugar + phosphoric acid residue), forming threads with a total length of about 2 meters. And we are not champions in this regard, because there are organisms whose DNA consists of hundreds of billions of nucleotides. In order for such a giant molecule to fit in the nucleus, invisible to the naked eye, DNA strands are twisted together into a helix (double helix) and wrapped around special proteins called histones. The cell has a special set of machines to work with this database.
You must constantly use the information contained in DNA: read the sequences that code for the proteins you currently need (transcription), and copy the entire database from time to time to divide the cell (replication). Each of these steps involves unraveling the helix of nucleotides. For this activity, the helicase enzyme is used, which moves in a spiral and - like a wedge - divides it into separate threads (all this resembles lightning). The enzyme works due to the energy released as a result of the breakdown of the universal energy carrier of the cell - ATP (adenosine triphosphate).
Model of the ATP molecule. Attachment and detachment of phosphate residues (left) provide energy exchange in cellular chemical reactions.
Now you can start copying chain fragments, which RNA polymerase does, also driven by the energy contained in ATP. The enzyme moves along the DNA strand and forms a region of RNA (containing sugar, ribose instead of deoxyribose), which is the template on which proteins are synthesized. As a result, DNA is preserved (avoiding constant unraveling and reading of fragments), and, in addition, proteins can be created throughout the cell, not just in the nucleus.
An almost error-free copy is provided by DNA polymerase, which acts similarly to RNA polymerase. The enzyme moves along the thread and builds up its counterpart. When another molecule of this enzyme moves along the second strand, the result is two complete strands of DNA. The enzyme needs a few "helpers" to start copying, tying fragments together, and removing unnecessary stretch marks. However, DNA polymerase has a "manufacturing defect". It can only move in one direction. Replication requires the creation of a so-called starter, from which the actual copying begins. Once completed, the primers are removed and, since the polymerase has no backup, it shortens with each DNA copy. At the ends of the thread are protective fragments called telomeres that do not code for any proteins. After their consumption (in humans, after about 50 repetitions), the chromosomes stick together and are read with errors, which causes cell death or its transformation into a cancerous one. Thus, the time of our life is measured by the telomeric clock.
Copying DNA requires many enzymes to work together.
A DNA-sized molecule undergoes permanent damage. Another group of enzymes, also acting as specialized machines, deals with troubleshooting. An explanation of their role was awarded the 2015 Chemistry Prize (for more information see the January 2016 article).
Inside…
… cells have a cytoplasm - a suspension of components that fill them with various vital functions. The entire cytoplasm is covered with a network of protein structures that make up the cytoskeleton. The contracting microfibers allow the cell to change its shape, allowing it to crawl and move its internal organelles. The cytoskeleton also includes microtubules, i.e. tubes made of proteins. These are fairly rigid elements (a hollow tube is always stiffer than a single rod of the same diameter) that form a cell, and some of the most unusual molecular machines move along them - walking proteins (literally!).
Microtubules have electrically charged ends. Proteins called dyneins move towards the negative fragment, while kinesins move in the opposite direction. Thanks to the energy released from the breakdown of ATP, the shape of walking proteins (also known as motor or transport proteins) changes in cycles, allowing them to move like a duck across the surface of microtubules. Molecules are equipped with a protein "thread", to the end of which another large molecule or a bubble filled with waste products can stick. All this resembles a robot, which, swaying, pulls a balloon by a string. Rolling proteins transport the necessary substances to the right places in the cell and move its internal components.
Almost all reactions occurring in the cell are controlled by enzymes, without which these changes would almost never occur. Enzymes are catalysts that act like specialized machines to do one thing (very often they only speed up one particular reaction). They capture the substrates of transformation, arrange them appropriately to each other, and after the end of the process they release the products and start working again. The association with an industrial robot performing endlessly repetitive actions is absolutely true.
Molecules of the intracellular energy carrier are formed as a by-product of a series of chemical reactions. However, the main source of ATP is the work of the most complex mechanism of the cell - ATP synthase. The largest number of molecules of this enzyme is located in the mitochondria, which act as cellular "power plants".
ATP synthase - top: fixed part
in the membrane, drive shaft, responsible fragment
for ATP synthesis
In the process of biological oxidation, hydrogen ions are transported from the inside of individual sections of the mitochondria to the outside, which creates their gradient (concentration difference) on both sides of the mitochondrial membrane. This situation is unstable and there is a natural tendency for concentrations to equalize, which is what ATP synthase takes advantage of. The enzyme consists of several moving and fixed parts. A fragment with channels is fixed in the membrane, through which hydrogen ions from the environment can penetrate into the mitochondria. Structural changes caused by their movement rotate another part of the enzyme - an elongated element that acts as a drive shaft. At the other end of the rod, inside the mitochondrion, another piece of the system is attached to it. The rotation of the shaft causes the rotation of the internal fragment, to which, in some of its positions, substrates of the ATP-forming reaction are attached, and then, in other positions of the rotor, a ready-made high-energy compound. released.
And this time it is not difficult to find an analogy in the world of human technology. Just an electricity generator. The flow of hydrogen ions makes the elements move inside the molecular motor immobilized in the membrane, like the blades of a turbine driven by a stream of water vapor. The shaft transfers the drive to the actual ATP generation system. Like most enzymes, synthase can also act in the other direction and break down ATP. This process sets in motion an internal motor which drives the moving parts of the membrane fragment through a shaft. This, in turn, leads to the pumping out of hydrogen ions from the mitochondria. So, the pump is electrically driven. Molecular miracle of nature.
On the border…
... Between the cell and the environment there is a cell membrane that separates the internal order from the chaos of the external world. It consists of a double layer of molecules, with the hydrophilic ("water-loving") parts outward and the hydrophobic ("water-avoiding") parts towards each other. The membrane also contains many protein molecules. The body has to come into contact with the environment: absorb the substances it needs and release waste. Some chemical compounds with small molecules (for example, water) can pass through the membrane in both directions according to the concentration gradient. Diffusion of others is difficult, and the cell itself regulates their absorption. Further, cellular machines are used for transmission - conveyors and ion channels.
The conveyor binds an ion or molecule and then moves with it to the other side of the membrane (when the membrane itself is small) or - when it passes through the entire membrane - moves the collected particle and releases it at the other end. Of course, conveyors work both ways and are very "finicky" - they often transport only one type of substance. Ion channels show a similar working effect, but a different mechanism. They can be compared to a filter. Transport through ion channels generally follows a concentration gradient (higher to lower ion concentrations until they level off). On the other hand, intracellular mechanisms regulate the opening and closing of passageways. The ion channels also exhibit high selectivity for particles to pass through.
Ion channel (left) and pipelines in operation
The bacterial flagellum is a true driving mechanism
There is another interesting molecular machine in the cell membrane - the flagellum drive, which ensures the active movement of bacteria. This is a protein engine consisting of two parts: a fixed part (stator) and a rotating part (rotor). Movement is caused by the flow of hydrogen ions from the membrane into the cell. They enter the channel in the stator and further into the distal part, which is located in the rotor. To get inside the cell, hydrogen ions must find their way to the next section of the channel, which is again in the stator. However, the rotor must rotate in order for the channels to converge. The end of the rotor, protruding beyond the cage, is curved, a flexible flagellum is attached to it, rotating like a helicopter propeller.
I believe that this necessarily brief overview of the cellular mechanism will make it clear that the winning designs of the Nobel Prize winners, without detracting from their achievements, are still far from the perfection of the creations of evolution.
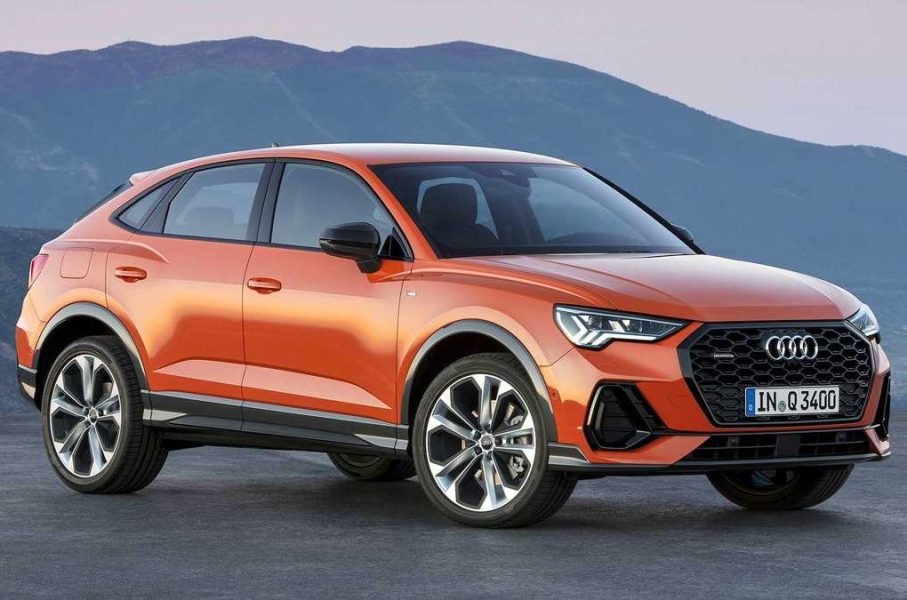
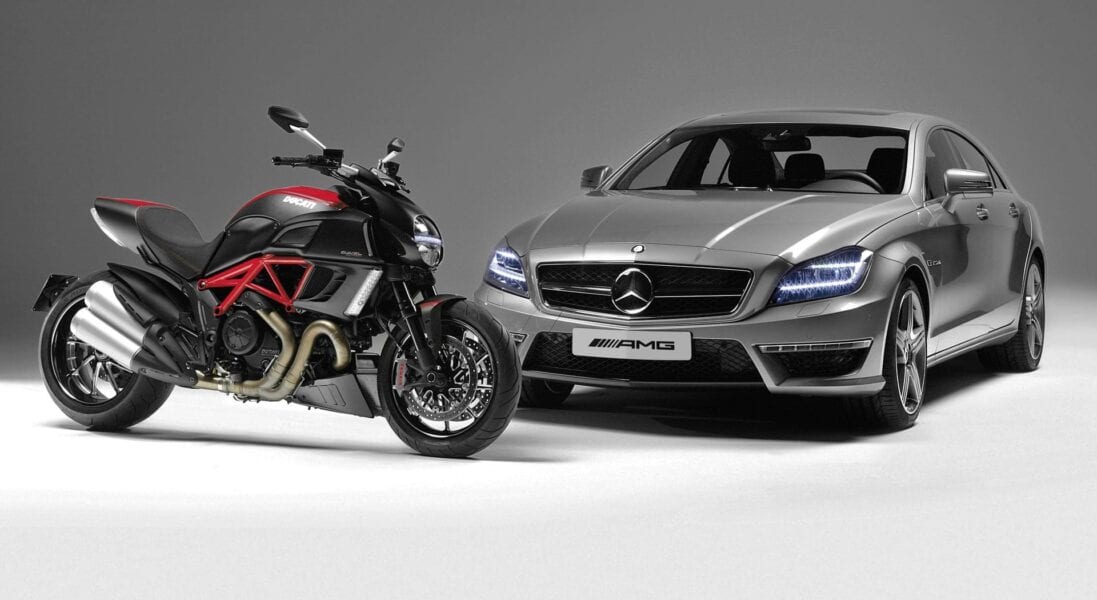