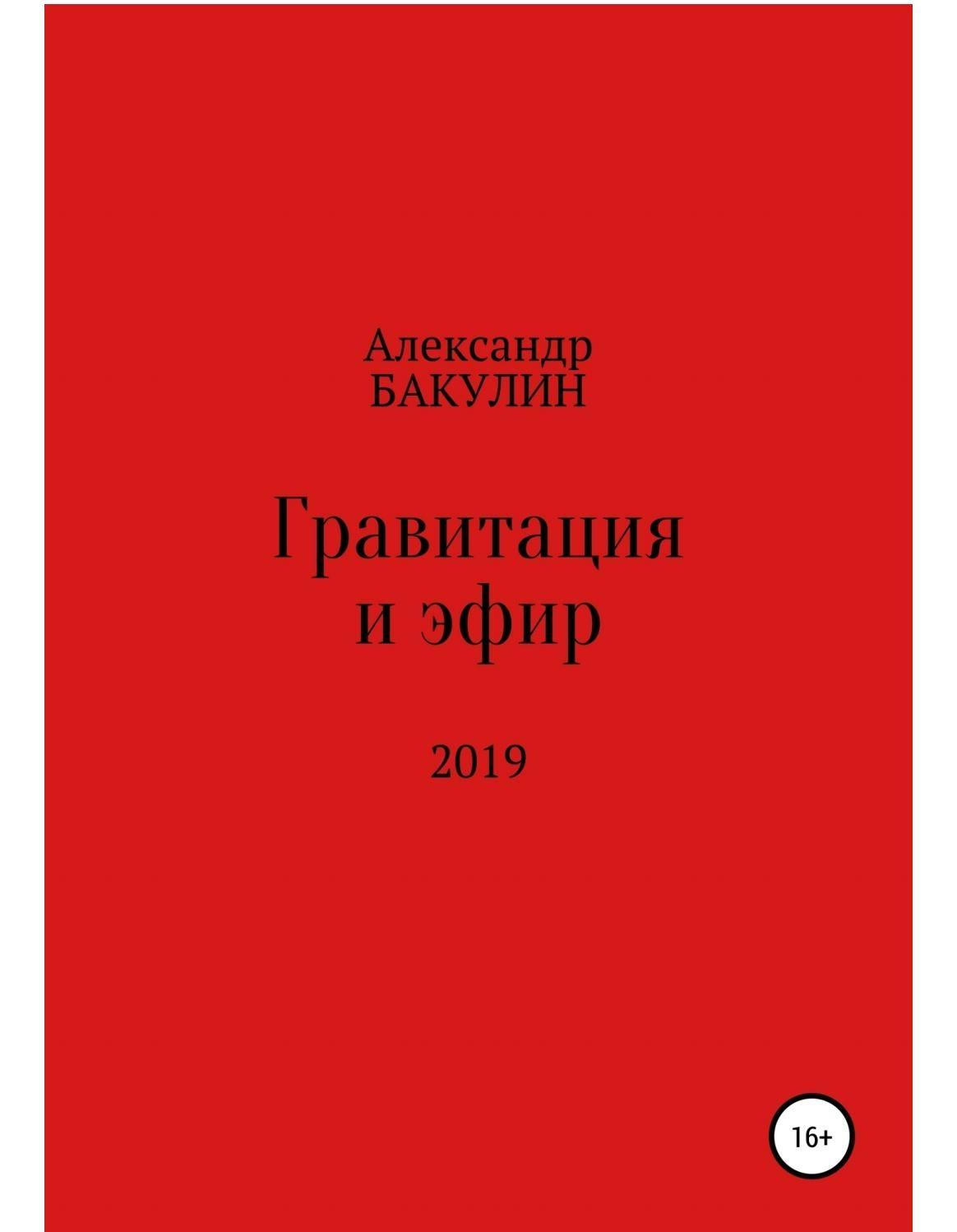
New physics shines through from many places
Content
Any possible changes we would like to make to the Standard Model of physics (1) or general relativity, our two best (though incompatible) theories of the universe, are already very limited. In other words, you cannot change much without undermining the whole.
The fact is that there are also results and phenomena that cannot be explained on the basis of the models known to us. So should we go out of our way to make everything inexplicable or inconsistent at any cost consistent with existing theories, or should we look for new ones? This is one of the fundamental questions of modern physics.
The Standard Model of particle physics has successfully explained all the known and discovered interactions between particles that have ever been observed. The universe is made up of quarks, leptonov and gauge bosons, which transmit three of the four fundamental forces in nature and give particles their rest mass. There is also general relativity, our, unfortunately, not a quantum theory of gravity, which describes the relationship between space-time, matter and energy in the universe.
The difficulty in going beyond these two theories is that if you try to change them by introducing new elements, concepts and quantities, you will get results that contradict the measurements and observations we already have. It's also worth remembering that if you want to go beyond our current scientific framework, the burden of proof is enormous. On the other hand, it's hard not to expect so much from someone who undermines models tried and tested for decades.
In the face of such demands, it is not surprising that hardly anyone tries to completely challenge the existing paradigm in physics. And if it does, it is not taken seriously at all, as it quickly stumbles on simple checks. So, if we see potential holes, then these are just reflectors, signaling that something is shining somewhere, but it is not clear whether it is worth going there at all.
Known physics can't handle the universe
Examples of the shimmer of this “completely new and different”? Well, for example, observations of the recoil rate, which seem inconsistent with the statement that the Universe is filled only with particles of the Standard Model and obeys the general theory of relativity. We know that individual sources of gravity, galaxies, clusters of galaxies, and even the great cosmic web are not enough to explain this phenomenon, perhaps. We know that, although the Standard Model states that matter and antimatter should be created and destroyed in equal amounts, we live in a universe composed mostly of matter with a small amount of antimatter. In other words, we see that "known physics" cannot explain everything we see in the universe.
Many experiments have yielded unexpected results that, if tested at a higher level, could be revolutionary. Even the so-called Atomic Anomaly indicating the existence of particles can be an experimental error, but it can also be a sign of going beyond the Standard Model. Different methods of measuring the universe give different values for the rate of its expansion - a problem that we considered in detail in one of the recent issues of MT.
However, none of these anomalies gives sufficiently convincing results to be considered an indisputable sign of new physics. Any or all of these may simply be statistical fluctuations or an incorrectly calibrated instrument. Many of them may point to new physics, but they can just as easily be explained using known particles and phenomena in the context of general relativity and the Standard Model.
We plan to experiment, hoping for clearer results and recommendations. We may soon see if dark energy has a constant value. Based on planned galaxy studies by the Vera Rubin Observatory and data on distant supernovae to be made available in the future. nancy grace telescope, previously WFIRST, we need to find out if dark energy evolves with time to within 1%. If so, then our "standard" cosmological model will have to be changed. It is possible that the space laser interferometer antenna (LISA) in terms of plan will also give us surprises. In short, we are counting on the observation vehicles and experiments that we are planning.
We are also still working in the field of particle physics, hoping to find phenomena outside the Model, such as a more accurate measurement of the magnetic moments of the electron and muon - if they do not agree, new physics appears. We're working to figure out how they fluctuate neutrino – here, too, new physics shines through. And if we build an accurate electron-positron collider, circular or linear (2), we can detect things beyond the Standard Model that the LHC cannot yet detect. In the world of physics, a larger version of the LHC with a circumference of up to 100 km has long been proposed. This would give higher collision energies, which, according to many physicists, would finally signal new phenomena. However, this is an extremely expensive investment, and the construction of a giant only on the principle - "let's build it and see what it will show us" raises a lot of doubts.
2. Linear lepton collider - visualization
There are two types of approach to problems in physical science. The first is a complex approach, which consists in the narrow design of an experiment or an observatory for solving a specific problem. The second approach is called the brute force method.who develops a universal, boundary-pushing experiment or observatory to explore the universe in a completely new way than our previous approaches. The first is better oriented in the Standard Model. The second allows you to find traces of something more, but, unfortunately, this something is not exactly defined. Thus, both methods have their drawbacks.
Look for the so-called Theory of Everything (TUT), the holy grail of physics, should be placed in the second category, since more often than not it comes down to finding higher and higher energies (3), at which the forces of nature eventually combine into one interaction.
3. Energies required for a hypothetical unification of interactions
Nisforn neutrino
Recently, science has become more and more focused on more interesting areas, such as neutrino research, on which we recently published an extensive report in MT. In February 2020, the Astrophysical Journal published a publication about the discovery of high-energy neutrinos of unknown origin in Antarctica. In addition to the well-known experiment, research was also carried out on the frosty continent under the code name ANITA (), consisting in the release of a balloon with a sensor radio waves.
Both and ANITA were designed to search for radio waves from high-energy neutrinos colliding with the solid matter that makes up ice. Avi Loeb, chairman of the Harvard Department of Astronomy, explained on the Salon website: “The events detected by ANITA certainly seem like an anomaly because they cannot be explained as neutrinos from astrophysical sources. (...) It could be some kind of particle that interacts weaker than a neutrino with ordinary matter. We suspect that such particles exist as dark matter. But what makes ANITA events so energetic?”
Neutrinos are the only known particles to violate the Standard Model. According to the Standard Model of elementary particles, we must have three types of neutrinos (electronic, muon and tau) and three types of antineutrinos, and after their formation they must be stable and unchanged in their properties. Since the 60s, when the first calculations and measurements of neutrinos produced by the Sun appeared, we realized that there was a problem. We knew how many electron neutrinos were formed in solar core. But when we measured how many arrived, we saw only a third of the predicted number.
Either something is wrong with our detectors, or something is wrong with our model of the Sun, or something is wrong with the neutrinos themselves. Reactor experiments quickly disproved the notion that something was wrong with our detectors (4). They worked as expected and their performance was very well rated. The neutrinos we detected were registered in proportion to the number of arriving neutrinos. For decades, many astronomers have argued that our solar model is wrong.
4. Images of neutrino events in Cherenkov radiation from the Super Kamiokande detector
Of course, there was another exotic possibility that, if true, would change our understanding of the universe from what the Standard Model predicted. The idea is that the three types of neutrinos we know actually have mass, not lean, and that they can mix (fluctuate) to change flavors if they have enough energy. If the neutrino is electronically triggered, it can change along the way to muon i taonovbut this is possible only when it has mass. Scientists are concerned about the problem of right- and left-handed neutrinos. For if you cannot distinguish it, you cannot distinguish whether it is a particle or an antiparticle.
Can a neutrino be its own antiparticle? Not according to the usual Standard Model. Fermionsin general they should not be their own antiparticles. A fermion is any particle with a rotation of ± XNUMX/XNUMX. This category includes all quarks and leptons, including neutrinos. However, there is a special type of fermions, which so far exists only in theory - the Majorana fermion, which is its own antiparticle. If it existed, something special might be happening... neutrino free double beta decay. And here is a chance for experimenters who have long been looking for such a gap.
In all observed processes involving neutrinos, these particles exhibit a property that physicists call left-handedness. Right-handed neutrinos, which are the most natural extension of the Standard Model, are nowhere to be seen. All other MS particles have a right-handed version, but neutrinos do not. Why? The latest, extremely comprehensive analysis by an international team of physicists, including the Institute of Nuclear Physics of the Polish Academy of Sciences (IFJ PAN) in Krakow, has done research on this issue. Scientists believe that the lack of observation of right-handed neutrinos could prove that they are Majorana fermions. If they were, then their right-sided version is extremely massive, which explains the difficulty of detection.
Yet we still don't know if neutrinos are antiparticles themselves. We don't know if they get their mass from the very weak binding of the Higgs boson, or if they get it through some other mechanism. And we don't know, maybe the neutrino sector is much more complex than we think, with sterile or heavy neutrinos lurking in the dark.
Atoms and other anomalies
In elementary particle physics, besides the fashionable neutrinos, there are other, less well-known areas of research from which "new physics" can shine through. Scientists, for example, have recently proposed a new type of subatomic particle to explain the enigmatic kaon decay (5), a special case of a meson particle consisting of one quark i one antique dealer. When kaon particles decay, a small fraction of them undergo changes that surprised scientists. The style of this decay may indicate a new type of particle or a new physical force at work. This is outside the scope of the Standard Model.
There are more experiments to find gaps in the Standard Model. These include the search for the g-2 muon. Nearly a hundred years ago, physicist Paul Dirac predicted the magnetic moment of an electron using g, a number that determines the spin properties of a particle. Then measurements showed that "g" is slightly different from 2, and physicists began to use the difference between the actual value of "g" and 2 to study the internal structure of subatomic particles and the laws of physics in general. In 1959, CERN in Geneva, Switzerland, conducted the first experiment that measured the g-2 value of a subatomic particle called a muon, bound to an electron but unstable and 207 times heavier than an elementary particle.
Brookhaven National Laboratory in New York started its own experiment and published the results of their g-2 experiment in 2004. The measurement was not what the Standard Model predicted. However, the experiment did not collect enough data for statistical analysis to conclusively prove that the measured value was indeed different and not just a statistical fluctuation. Other research centers are now doing new experiments with g-2, and we will probably know the results soon.
There is something more intriguing than this Kaon anomalies i muon. In 2015, an experiment on the decay of beryllium 8Be showed an anomaly. Scientists in Hungary use their detector. However, incidentally, they discovered or thought they discovered, which suggests the existence of a fifth fundamental force of nature.
Physicists from the University of California became interested in the study. They suggested that the phenomenon called atomic anomaly, was caused by a completely new particle, which was supposed to carry the fifth force of nature. It is called X17 because its corresponding mass is thought to be nearly 17 million electron volts. This is 30 times the mass of an electron, but less than the mass of a proton. And the way X17 behaves with a proton is one of its strangest features - that is, it does not interact with a proton at all. Instead, it interacts with a negatively charged electron or neutron, which has no charge at all. This makes it difficult to fit the X17 particle into our current Standard Model. Bosons are associated with forces. Gluons are associated with the strong force, bosons with the weak force, and photons with electromagnetism. There is even a hypothetical boson for gravity called a graviton. As a boson, X17 will carry a force of its own, such as that which until now has been a mystery to us and could be.
The universe and its preferred direction?
In a paper published this April in the journal Science Advances, scientists at the University of New South Wales in Sydney reported that new measurements of light emitted by a quasar 13 billion light-years away confirm previous studies that found small variations in the fine constant structure of the universe. Professor John Webb from UNSW (6) explains that the fine structure constant "is a quantity that physicists use as a measure of the electromagnetic force." electromagnetic force maintains electrons around the nuclei in every atom in the universe. Without it, all matter would fall apart. Until recently, it was considered a constant force in time and space. But in his research over the past two decades, Professor Webb has noticed an anomaly in the solid fine structure in which the electromagnetic force, measured in one chosen direction in the universe, always seems to be slightly different.
"" explains Webb. The inconsistencies appeared not in the Australian team's measurements, but in comparing their results with many other measurements of quasar light by other scientists.
"" says Professor Webb. "". In his opinion, the results seem to suggest that there may be a preferred direction in the universe. In other words, the Universe would in some sense have a dipole structure.
"" Says the scientist about the marked anomalies.
This is one more thing: instead of what was thought to be a random spread of galaxies, quasars, gas clouds and planets with life, the universe suddenly has a northern and southern counterpart. Professor Webb is nevertheless ready to admit that the results of measurements by scientists carried out at different stages using different technologies and from different places on the Earth are in fact a huge coincidence.
Webb points out that if there is directionality in the universe, and if electromagnetism turns out to be slightly different in certain regions of the cosmos, the most fundamental concepts behind much of modern physics will need to be revisited. "", speaks. The model is based on Einstein's theory of gravity, which explicitly assumes the constancy of the laws of nature. And if not, then ... the thought of turning the whole edifice of physics is breathtaking.
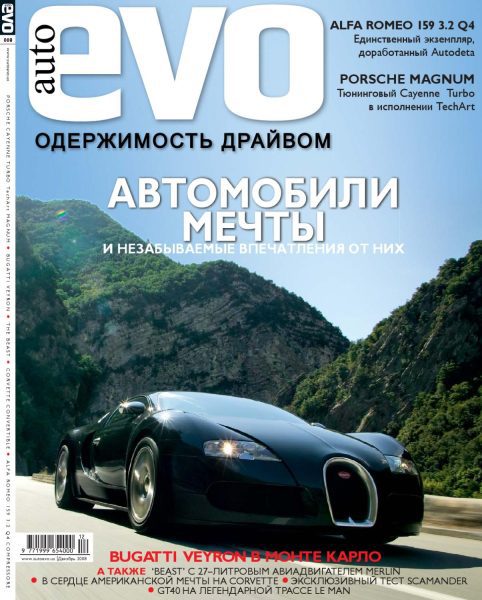
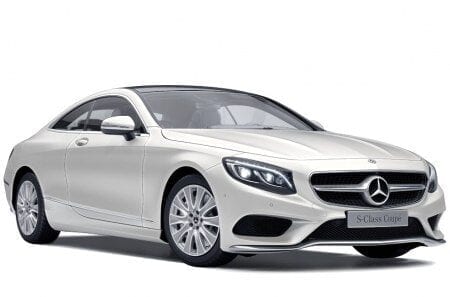